Regarding Uncertainty
As described in the section on Quantification of Uncertainty, it is
important to note that only a limited part of the total uncertainty
associated with future climate change is covered by the NARCCAP
simulations. Only one emissions scenario and four AOGCMs are used. A
total of 23 different AOGCMs participated in the Fourth Assessment
Report of the IPCC and are available at the PCMDI web site. However,
based on earlier work (e.g., Tebaldi et al., 2005) the climate changes
simulated by the four AOGCMs can be placed in the context of
probabilities of future climate change generated using the full suite
of climate models. This will allow NARCCAP results to be placed in the
context of the large number of AOGCM results. The uncertainty related
to spatial scale is relatively well-covered since six different
regional models are used. As always, however, uncertainties that
cannot be readily quantified through use of even the full suite of
global climate models still must be recognized. These include such
uncertainties as processes that are missing from the climate models
(e.g., a fully coupled carbon cycle) processes that are not understood
well enough to model successfully (e.g., certain aspects of ice sheet
dynamics), and processes that we are still unaware of.
Regarding the Data
The data from the climate model simulations are provided as-is. We
have implemented quality control procedures to correct any errors
occurring in the creation of the data sets, but these procedures are
not perfect. In addition, data corruption can occur during storage and
download. When errors are found in published data, we correct them and
attempt to notify those users who have downloaded the affected
files.
Note also that the NCEP-driven simulations and current climate
simulations using boundary conditions from the AOGCMs do contain
biases compared to observed data for the same time periods. Climate
model simulations are not perfect. We will, however, provide some
insights into the credibility of the future climate simulations
through detailed analysis of the model results, including an
assessment of the quality of the current model simulations and their
representation of processes which are responsible for the projected
climate changes.
Regarding Time
Published NARCCAP data includes data from the initial equilibration
or 'spin-up' period of the run. For general use, we strongly
recommend that spin-up data be excluded for analysis, and that only
data from 1980-2004 (NCEP), 1971-2000 (GCM current), or 2041-2070
(future) be used. For more details, see the Time Periods Covered page.
Regarding WRF Simulations nested in the
CCSM: Treatment of the Great Lakes and other smaller lakes resolved by
WRF at 50 km grid resolution.
- For the current climate simulations, lake temperatures were
assigned in the standard fashion by spatially interpolating the CCSM
sea surface temperatures (SSTs) from coast to coast (Atlantic/Pacific)
to the locations of the lakes. This is a fairly standard practice
used by many of the regional models. Lake ice forms when the assigned
lake temperature is below 271.4K (-1.6° C) or when sea ice is
identified by the sea ice mask from CCSM.
- For the future simulation, lake temperatures were inadvertently
assigned based on the CCSM skin (i.e. surface) temperature at the
locations of the lakes. Lake ice forms when the assigned lake
temperature is below 271.4K (-1.6° C).
- However, the surface package of the CCSM (the Community Land
Model, CLM) includes a lake thermodynamics model to simulate subgrid
lakes. For larger lakes such as Lake Superior, the CCSM grid cell mean
surface temperatures are dominated by the surface temperatures
simulated for the lake surface type. Therefore, the lake temperature
assigned based on the CCSM skin temperature is reflective of the lake
surface type and close to the temperature of the lakes. However, this
is less true for the smaller lakes (Ontario and Erie) for which the
subgrid percent lake is lower than 50%; for Ontario in particular it
is only between 20 and 30%.
- Since lake temperatures in the future simulation were not
correctly assigned, we recommend that near surface variables such as
surface temperature and surface heat fluxes, over the lake points for
the two smaller lakes of the Great Lakes and other small lakes such as
those in the Canadian prairie, in the future simulation not be used in
analysis of the model outputs.
Rerun of the RegCM3 using GFDL driving data:
Improvement in the treatment of the Great Lakes (but sea ice problem
remains)
- For the original GFDL-driven current and future simulations,
initial lake temperatures were assigned using downward-extrapolated
air temperature for the GFDL land points corresponding to the lake
point locations in the RegCM3. After the initial time step, the BATS
land surface model in RegCM3 calculates the temperatures at lake grid
points. Grid points designated as lakes use the BATS parameterization
for inland water (land use category 14). This land type does not
correspond well to the characteristics of a lake, and so the lake
temperatures, particularly in summer, do not correspond well to what
one would expect for lake temperatures compared to surrounding land.
For example, in summer the lake temperatures should be cooler than
that of surrounding land. This is not the case in the original RegCM3
simulations.
To correct for this situation, the RegCM3 model was rerun using the
GFDL model boundary conditions, but using a different means of
representing the lakes. As is done in a number of other regional
climate models, the lake temperatures were determined by interpolating
for the same latitudes the sea surface temperatures of the adjacent
oceans to the locations of the Great Lakes grids (this is a weighted
interpolation using both Atlantic and Pacific Ocean SSTs). The lake
surface temperatures in the simulations are now more realistic. The
adjacent figures show the surface temperatures in the Great Lakes
regions for winter and summer from the simulations of the current and
future periods for a) the old runs and b) the new set of runs.
Another limitation is that lake ice does not form in either run. So
both sets of simulations have limitations in how accurately they
portray the winter and early spring seasons over the lakes
themselves. For further reference, the RegCM3 source code and
documentation are available here: http://users.ictp.it/RegCNET/model.html.
Current | Future |
a) Original GFDL run |
DJF |
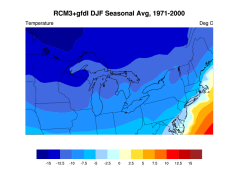 |
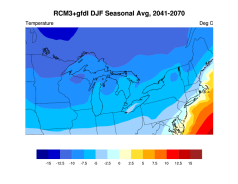 |
---|
JJA |
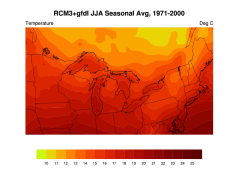 |
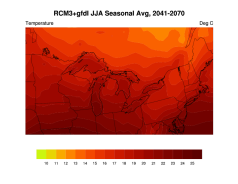 |
---|
b) GFDL rerun |
DJF |
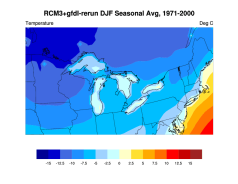 |
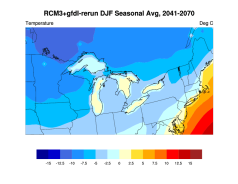 |
---|
JJA |
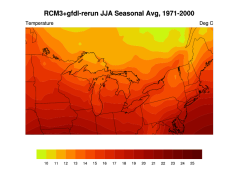 |
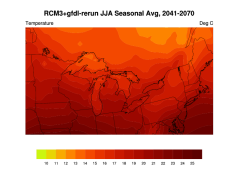 |
- In the GFDL future and historical simulations, sea ice on ocean
points (BATS land use category 15) does not form, due to problems with
RegCM3's sea ice code. The temperatures of those grid points are set
to the temperatures from the GCM SSTs.
- Note that there are no problems in the original simulations for
all other parts of the NARCCAP domain and they can be used. There is
little difference in the two runs for these other parts of North
America.
Caveat regarding RegCM3 simulations using CGCM3 driving data: Treatment of the Great Lakes.
- For the CGCM3 future and historical simulations, initial lake temperatures were assigned using downward-extrapolated air temperature. After the initial time step, the BATS land surface model calculates the temperatures at lake grid points. Grid points designated as lakes use the BATS parameterization for inland water (land use category 14). See the description of this land use category below.
- CGCM3 surface temperature data includes two (?) lake points, from the lake model within CGCM3, and these temperatures are incorporated into RegCM3.
- Users should carefully evaluate the model output for the Great Lakes region to determine if it is suitable for their uses. Surface variables should be considered suspect.
- In the CGCM future and historical simulations, sea ice on ocean points (BATS land use category 15) does not form, due to problems with RegCM3's sea ice code. The temperatures of those grid points are set to the temperatures from the GCM SSTs.
About BATS land use category 14 (inland water)
In the BATS land surface model, used by RegCM3, lake grid cells are defined as land use category 14. This land use type is treated in the same way as any other land use type by the land surface model (i.e. fluxes of heat and moisture are calculated, along with other variables). The response of land use category 14 is governed by the parameters in the tables below. The land surface model also keeps category 14 saturated at all time steps, by setting negative values for runoff. This is the best approximation for a water covered grid cell given BATS limitations. For further reference, the RegCM3 source code and documentation are available here.
BATS land use category 14 (inland water) parameters. Obtained from BLOCKDATA001.F in RegCM3 source code.
Parameter name and description |
Parameter value |
vegc - maximum fractional cover of vegetation: | 0 |
seasf - difference between vegc and fractional cover at 269K: | 0 |
rough - aerodynamic roughness length (m): | 0 |
displa - displacement height (m): | 0 |
albvgs - vegetation albedo for wavelength lt 0.7 microns: | 0.07 |
albvgl - vegetation albedo for wavelengths ge 0.7 microns: | 0.20 |
rsmin - min stomatal resistance (s/m): | 200 |
xla - max leaf area index: | 0 |
xlai0 - min leaf area index: | 0 |
sai - stem area index: | 2 |
sqrtdi - inverse square root of leaf dimension: | 5.0 |
fc - light dependence of stomatal resistance: | .02 |
depuv - depth of upper soil layer (mm): | 100. |
deprv - depth of root zone (mm): | 1000. |
deptv - depth of total soil (mm): | 3000. |
iexsol - soil texture type: | 6 |
kolsol - soil color type: | 5 |
Parameters specific to soil texture type 6, from BLOCKDATA001.F in RegCM3 source code (note: in the case of BATS land use category 14, only the saturated albedos are applicable since these grid cells are saturated at all times).
Parameter name and description | Parameter value |
xmopor - fraction of soil that is voids (porosity): | 0.48 |
xmosuc - min soil suction (mm): | 200 |
xmohyd - saturated hydraulic conductivity (mm s-1): | 6.3 x 10-3 |
xmowil - moisture content relative to saturation at which transpirations ceases: | .332 |
xmofc -fraction of water content at which wilting occurs: | .688 |
bee - clapp and hornberger (1978) "B" exponent: | 6.0 |
skrat - ratio of saturated thermal conductivity to that of loam: | 1.0 |
dry soil albedo for wavelengths lt 0.7 microns: | 0.16 |
Dry soil albedo for wavelengths ge 0.7 microns: | 0.32 |
saturated soil albedo for wavelengths lt 0.7 microns: | 0.08 |
saturated soil albedo for wavelengths ge 0.7 microns: | 0.16 |
Caveat regarding HRM3 Treatment of Great Lakes Temperatures in the GFDL-Driven Runs.
There was no GFDL SST or sea ice data available for the Great Lakes.
For the current-period experiment, which was simulated first, monthly
climatology values were used. However, when it came to starting the
future period (scenario) experiment, climatological values were not
suitable.
For the scenario run, the modelers used the SST ancillaries from
the HadCM3Q0 scenario simulation to calculate the monthly climatology
difference in SSTs between each grid box in the great lakes and a
nearby ocean grid box from James Bay. This difference was then applied
to the GFDL data to create SSTs over the great lakes. The sea ice
fraction for the Great Lakes was set to zero.
The lake temperatures for the two runs thus come from two
independent sources, and this inconsistency allows the future period
summer lake temperatures to be cooler than the current period summer
lake temperatures.
References
Tebaldi, C., R. Smith, D. Nychka, and L. O. Mearns, 2005:
Quantifying uncertainty in projections of regional climate change: A
Bayesian approach to the analysis of multi-model ensembles.
J. Climate 18:1524-1540.
|